'Spectacle' Array of 210Po Halo Radiocentres in Biotite: a Nuclear Geophysical Enigma
(reprinted from Nature, Vol. 252, No. 5484, pp. 564−566, December 13, 1974)
Polonium radiohaloes occur widely and not infrequently
(total about 1015−l020) in Precambrian rocks but their existence
has so far defied satisfactory explanation based on accepted
nucleocosmogeochemical theories1. Do Po haloes imply that
unknown processes were operative during the formative period
of the Earth? Is it possible that Po halos in Precambrian rocks
represent extinct natural radioactivity2 and are therefore of
cosmological significance? A detailed comparison between an
unusual array of Po halo radiocentres and U−Th halo
radiocentres is presented here as bearing on the above questions.
Generally, radiohaloes occur in one of several mineralogical
contexts1, 3, 4.
First, as single haloes around discrete inclusions
well isolated from other mineral defects and haloes; second, as
single haloes around discrete inclusions lodged in conduits or
cleavage cracks; third, as single haloes randomly spaced in
clusters (sometimes overlapping); fourth, as vein haloes which
formed from a continuous distribution of radioactivity (apparently
deposited from hydrothermal solutions) along a conduit;
and fifth, as line haloes, which surround, not conduits or cracks,
but genuine single inclusions which are long (for example, 25
μm) compared with their width (perhaps 1 μm). Large,
amorphous, coloured regions without discrete inclusions are not
haloes.
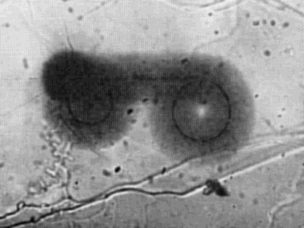 | Fig. 1 'Spectacle' array of 210Po haloes in biotite. Halo
radius, 18.5 μm. |
A striking exception1 to this classification is the 'spectacle'
coloration pattern (Fig. 1), which exhibits two almost circular
rings of inclusions joined by a linear array of inclusions. As
far as we know this is unlike any group of haloes previously
seen. This geometrical arrangement of halo radiocentres, found
in a Precambrian biotite from Silver Crater Mine, Faraday
Township, Ontario, exhibits true radiohalo characteristics.
First, the coloration is identical to that of normal haloes found
about 300 μm away in the same mica specimen. Second, the
three-dimensional nature of the halo pattern was demonstrated
when the specimen (initially about 50 μm thick) was cleaved;
both halves revealed matching 'spectacle' coloration patterns,
the only difference being the presence of the inclusion array in
one half and its absence in the other half. Third, the radius of the
coloration band (18.5 μm) implied an origin from 210Po α decay.
Mass spectrometric and X-ray fluorescence methods were used
to ascertain whether this was indeed a Po halo array.
Before applying these techniques to the 'spectacle' halo, we
established that ion-microprobe mass analyses and scanning
electron microscope X-ray fluorescence (SEMXRF) studies of
'normal' or 'standard' halo radiocentres (those formed from
both U and Th α decay) yielded data consistent with the visual
means of identification. Several U−Th haloes (see, for
example, photo insert, Fig. 2)
found in a Precambrian pegmatitic
mica from Rossi, New York, were analyzed by X-ray and ion-probe
techniques. Several U−Th halo radiocentres were chosen
which contained only U, Th and Pb in any significant
abundance, thereby virtually eliminating any molecular ion
interference in the Pb−Th−U region (m/e = 204−238) in the
ion probe.
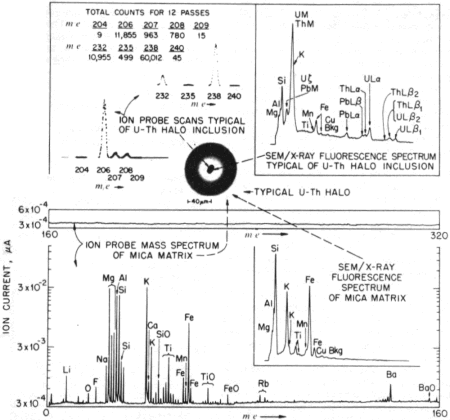 | Fig. 2 Ion microprobe
and XRF comparison between mica matrix and U−Th halo inclusion. |
That the mica matrix5 yielded insignificant molecular ion
currents in the region m/e 160−320 is evident from the data in
the lower portion of Fig. 2. In contrast, the recorded spectra of a
U−Th inclusion (upper left portion of Fig. 2) revealed a
significant number of ion counts accumulated in 12 passes of the
regions m/e 204−209 and (with a different scale) m/e 232−240.
Total ion counts are tabulated just above the two spectra.
The scans on the Pb−Bi region (m/e 204−209) lasted for several
minutes and were taken before the scans (equal time) on the U−Th region.
Exact 206Pb/238U and 208Pb/232Th
ratios are not obtainable
from the ion count data in Fig. 2 because variable U and Th concentrations
were observed as the ion probe beam sputtered away
the inclusion; accurate ratios could be obtained by simultaneously
accumulating counts in the region 204−238 provided
that the greater secondary ion yield of U and Th as compared
with Pb is taken into account. On the other hand, the separate Pb
and U isotope ratios are meaningful. Note, for example, that
after subtraction of background counts at m/e 240 from the total
counts at m/e 235 and 238, the 235/238 value (0.76) satisfactorily
approximates (considering the relatively small number
of counts collected) the natural U isotopic ratio, 235U/238U =
0.72. The absence of a peak at 204 shows there is little or no
common lead in the inclusion and therefore, that the 206/207
ratio is that of 206Pb/207Pb as derived from in situ U decay.
Also shown in Fig. 2 are the SEMXRF spectra of the mica
matrix and the U−Th halo radiocentre, both of which correlate
well (with the exception of the low Z and low abundance
elements in the former) with the respective ion-probe spectra.
Only U, Th and Pb are exclusively in the inclusion.
The ion-microprobe mass spectrum of the mica matrix surrounding
the 'spectacle' halo was nearly identical to the mica
spectrum shown in Fig. 2 and is not repeated in Fig. 3. Figure 3
(top centre) shows the portion m/e = 160−264 of the
ion-microprobe
spectrum (verical log scale) of several of the inclusions.
Also shown is the actual ion-probe trace of the important
region from m/e = 204−210 using a linear vertical scale and an
expanded horizontal scale. There is no significant ion current
above m/e = 209; that is, no significant ion signals were detected
at any of the prominent U and Th peaks: 238(U+), 254(UO+),
232(Th+) and 248(ThO+). No m/e = 204 was detected above
background (1 c.p.s.), and the 206/207 mass ratio was
≃20 (206
signal ≃2,000 c.p.s.).
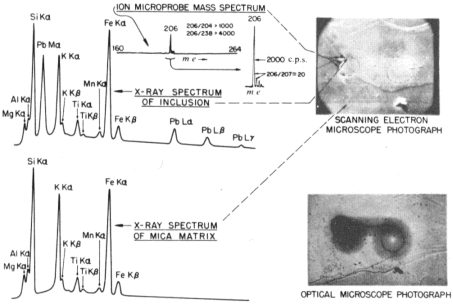 | Fig. 3 Ion microprobe
and SEMXRF spectra of mica matrix and 210Po halo inclusions. |
Figure 3 also shows SEMXRF spectra of the surrounding
mica and of one of the Po halo radiocentres. Lead is the only
element detectable in this radiocentre exclusive of the mica;
some adjacent radiocentres revealed Bi as well. The use of two
different instruments, and longer counting times, account for the
slightly different X-ray spectra in Figs 2 and 3. The excellent
resolution of the SEM showed the Pb-rich areas to coincide
exactly with the Po halo radiocentres which are visible both
in ordinary transmitted (Fig 1) and reflected light microscopy.
Regions as close as 1 μm to the radiocentres showed virtually no
Pb or Bi, implying little if any diffusion loss from the inclusions.
As the X-ray data definitely show Pb (and sometimes Bi) in
the 'spectacle' halo radiocentres, and as there is no evidence for
any molecular ion contribution in the region from m/e =
204−238, the 206, 207 and 208 peaks are interpreted as Pb isotopes and 209 as
209Bi. 204Pb, a constituent of both common and
primordial Pb, is missing (no 204 peak), implying that the 'spectacle'
halo inclusions analyzed contained no detectable Pb of either of these types.
Absence of the 232, 235 and 238 peaks is interpreted as showing the
inclusions contain virtually no 232Th, 235U or
238U and, therefore, no radiogenic 208Pb,
207Pb or 206Pb derived from the in situ
decay of these isotopes. The 207 and 208 peaks
are therefore attributed to 207Pb and 208Pb, perhaps arising from
the decay of minute amounts of 211Bi and 212Bi within the
inclusions5,6. The 209Bi is considered to be primordial.
The outstanding feature of the mass analysis is the prominent
206 signal which, when attributed to the presence of 206Pb in the
inclusions, fits perfectly with the prediction based on ring
structure measurements, that is, that the 206Pb is radiogenically
derived, not from U or Th, but directly from 210Po α decay. In
this respect, the large difference in the 206/238 (206Pb/236U) ratio
between the 'spectacle' halo and the U−Th halo (Figs 2 and 3)
is especially significant. Clearly the 'spectacle' halo resulted
from 210Po α decay; an explanation for its geometry is still under
study.
Because the Pb isotope in these inclusions is not explicable as
any combination of common, primordial, or from in situ Pb
derived radiogenically in situ from U or Th, we conclude that a
different type of Pb, derived from Po α decay, exists in nature.
Supportive evidence comes from electron-probe and ion-probe
analyses of a 218Po halo radiocentre found in a mica from the
Iveland District, Norway, which yielded a 206Pb/207Pb ratio of
23. This is consistent with that expected from 218Po a decay to
206Pb. Such a Pb ratio is impossibly high based on normal
isotopic 238U/235U decay, the theoretical maximum being 21.8.
Other investigations have shown varying mixtures of U-derived
and Po-derived Pb may occur in the same radiocentre,
for there exists an almost continuous halo spectrum stretching
from "pure" U to "pure" Po haloes. Only a few (<0.01) Po haloes
in biotite may survive the delicate sectioning process necessary
for SEM X-ray analysis.
Just as important as the existence of a new type of lead is the
question of whether Po haloes which occur in a granitic or pegmatitic
environment (for example, in mica, fluorite or cordierite)
can be explained by accepted models of Earth history1. (R. V. G.
has found other 210Po haloes that differ essentially from those in
granites-unpublished information.)
This research has been sponsored by the United States
Atomic Energy Commission under contract with Union Carbide
Corp. and by Columbia Union College with an assistance grant
from the National Science Foundation. Thanks are due to R. I.
Gait and J.A. Mandarino, Royal Ontario Museum, Louis Moyd,
National Museum of Canada, and G. Switzer, United States
National Museum, for providing specimens.
ROBERT V. GENTRY |
Chemistry Division,
Oak Ridge National Laboratory, |
L. D. HULETT |
Analytical Chemistry Division,
Oak Ridge National Laboratory |
S. S. CRISTY
J. F. MCLAUGHLIN |
Laboratory Development Department,
Oak Ridge Y-12 Plant,
Oak Ridge, Tennessee 37830 |
J. A. MCHUGH |
Knolls Atomic Power Laboratory,
Schenectady, New York 12301 |
MICHAEL BAYARD |
McCrone Associates,
2820 Michigan Street,
Chicago, Illinois 60616
|
Received July 31, 1974.
References
Gentry, R. V., Science, 184, 62 (1974).
Kohnan, T. P., Ann. N. Y. Acad Sci., 62, 503 (1956).
Henderson, G. H., Mushkat, C. M., and Crawford, D. P., Proc.
R. Soc., A158, 199 (1934); Henderson, G. H., and Turnbull,
L. G., ibid., 145, 582 (1934); Henderson, G. H., and Bateson,
S., ibid., 573 (1934).
Gentry, R. V., Ann. Rev. NucI. Sci., 23, 347 (1973).
Gentry, R. V., Cristy, S. S., McLaughlin, J. F., and McHugh, J.
A., Nature, 244, 282 (1973).
Gentry, R. V., Science, 173, 727 (1971).
|